Self-organization
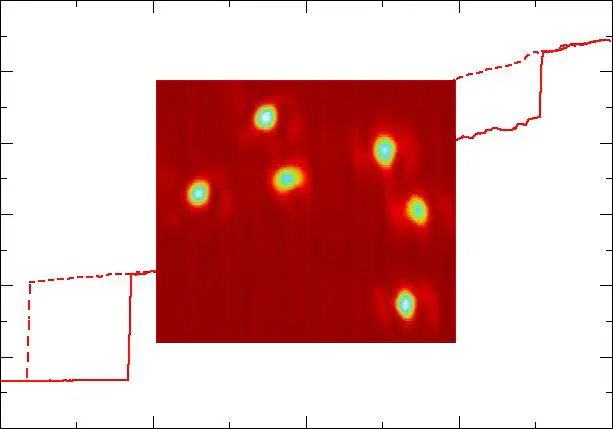
What is the reason that an initially homogeneous system evolves spontaneously into a modulated, “structured” state?
This intriguing question arises in many sciences, in nature as well as in the laboratory. Spontaneous self-organization phenomena in space and time are also ubiquitous in optical systems in which intense laser beams interact with a nonlinear medium, i.e. a medium in which the optical properties (refractive index or absorption coefficient) depend on the intensity of the incident light. The interplay of spatial coupling by diffraction and nonlinearity is responsible for the pattern formation. It is highly fascinating that the properties of structures in such different regions of science like hydrodynamics, chemical reactions, gas discharges and optics possess remarkably universal aspects. This spontaneous emergence of nontrivial – often highly ordered – states is very common in spatially extended systems driven out of thermal equilibrium.
Optics is promoting the knowledge on these dissipative patterns by demonstrating phenomena not known before. Therefore the investigation of optical patterns is an important topic of interdisciplinary research using equipment of technical relevance and might on the other hand form the basis for future all-optical data processing.
Nonlinear effects occur in many media and in many different configurations. Previous investigations in “hot” sodium vapor established well controlled nonlinear optical systems as ideal candidates for investigating principles of self-organization. The experiments yielded first demonstrations of phenomena predicted to occur in a variety of model and experimental systems as well as unprecedented phenomena enriching Nonlinear Science (see here for details). Beside technical advantages (high optical quality, easy variation of parameters over a broad range, high resonant nonlinearity) the benefit of using an atomic vapour is that the equations governing the light-matter interaction can be derived directly from quantum mechanics via the density matrix approach.
The essential new ingredient in cold atoms is that refractive index modulations are not only due to internal degrees of freedom of the atoms but that opto-mechanical coupling can lead to density modulations: The modulated light field causes dipole forces on the cold atoms, which will respond by transverse bunching. Depending on parameters, the opto-mechanical coupling can trigger feedback effects either damping or enhancing the modulation and induce new coupled light-matter instabilities. Spontaneous bunching of atoms in the longitudinal direction (i.e., along the wavevector of the laser beam) due to recoil and dipole effects is known from collective atomic recoil lasing (CARL, see the investigations in the CNQO group). However, the two-dimensional patterns sought after are much richer than CARL and other one-dimensional longitudinal wavelength-scale density modulations known to develop in cold atoms. If longitudinal CARL effects turn out to co-exist with the transverse instability, the resulting 3D self-organization would be a novel and highly significant result.